Nano computers are incredibly tiny devices, typically operating at the nanoscale, which is on the order of billionths of a meter. So why are these computers the talk of the scientific world? How will they shape our future?
Over the past four decades, electronic computers have witnessed remarkable advancements in terms of power and performance, largely driven by the shrinking size of their fundamental building block, the transistor. However, the relentless march towards smaller and smaller transistors is approaching a critical juncture due to the limitations imposed by quantum mechanics and fabrication techniques. Experts predict that once the transistor’s features shrink below 0.1 micrometers (or microns), these devices will no longer deliver practical functionality.
To overcome this barrier and continue the path of miniaturization down to the molecular scale, a paradigm shift is necessary. The existing designs of microelectronic devices must give way to novel approaches that harness the dominant influence of quantum mechanical effects at such minuscule dimensions. We invite you to enter the realm of nano computers, a groundbreaking field that explores the representation and manipulation of data through computers significantly smaller in size.
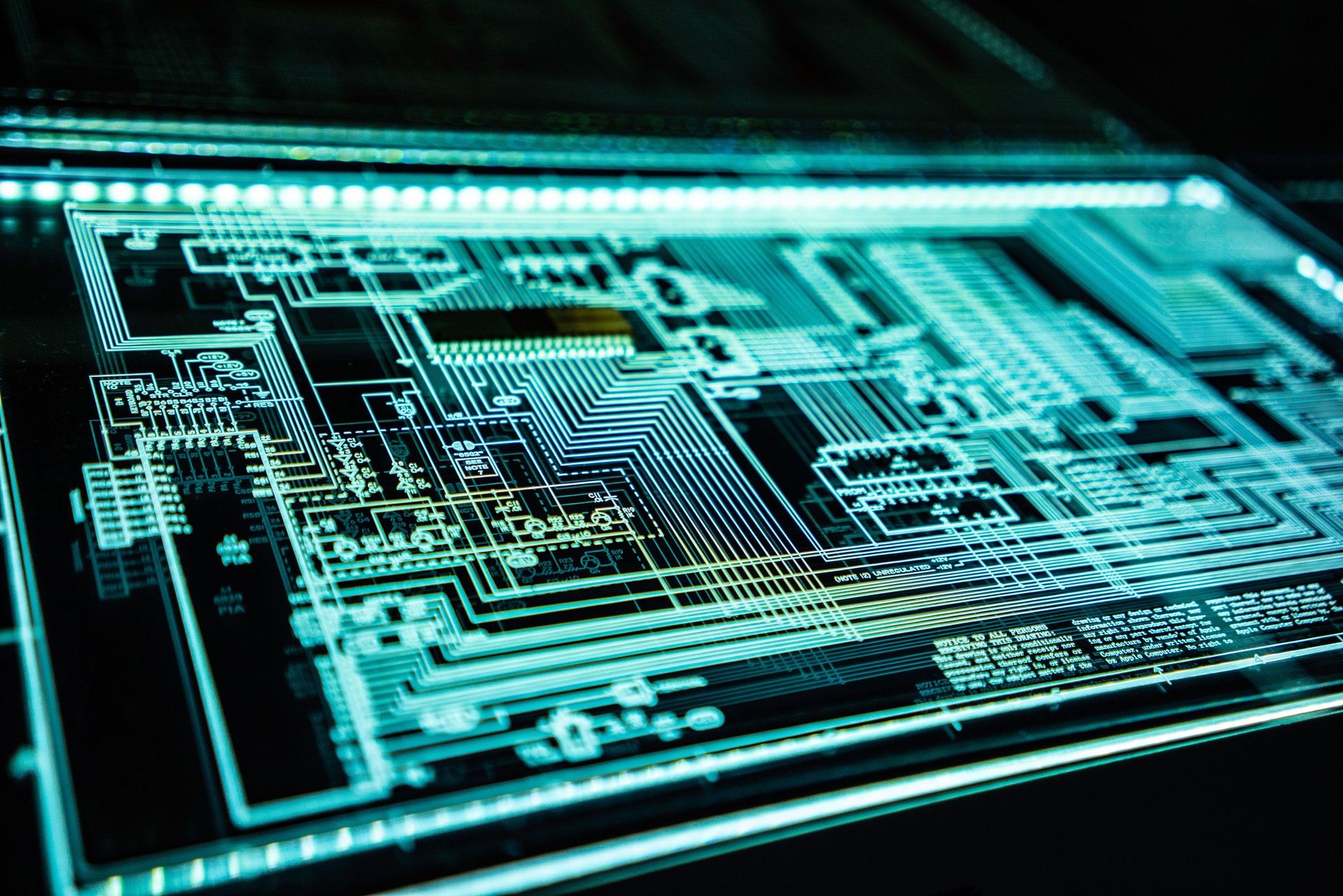
What are nano computers?
Nano computers are set to revolutionize computing by offering significantly smaller devices capable of data representation and manipulation. These tiny machines function similarly to modern personal computers but on a miniature scale. With the escalating demand for computing power, nanocomputers emerge as a cost-effective solution to extend computational capabilities.
Today, existing devices already employ transistors with channels measuring below 100 nanometers, driving researchers and scientists to strive for computers smaller than 10 nanometers. As this technology progresses, the future holds the promise of unbelievably compact nanocomputers that simplify computational tasks.
The minute size of nanocomputers necessitates the use of microscopes to observe them. These diminutive devices operate by storing data in quantum dots or spins. Their tiny form factor enables seamless integration into diverse environments, including the human body.
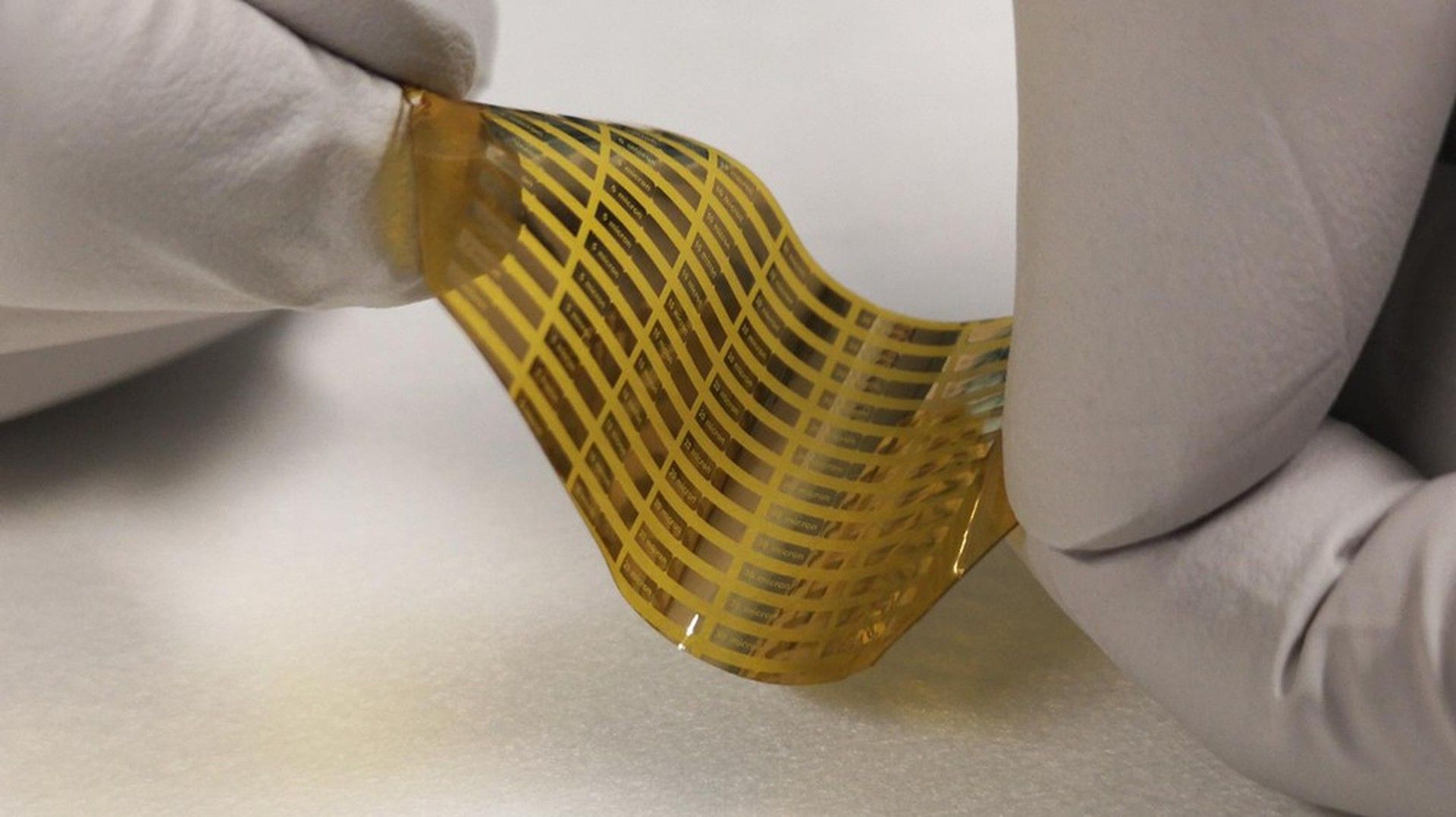
Leveraging their enhanced computational power and capabilities, nanocomputing technology finds extensive applications in the healthcare industry, unlocking new possibilities for improved diagnostics, monitoring, and treatment, just like the usage of artificial intelligence in healthcare.
However, it is important to approach nanocomputing with caution, as the technology is still in the developmental phase. Researchers and scientists continue to grapple with numerous challenges and limitations in realizing the full potential of nanocomputers.
As this groundbreaking field evolves, careful consideration must be given to factors such as scalability, reliability, and potential ethical implications, ensuring that nanocomputers are harnessed responsibly and securely in various domains.
Understanding the term ”nano”
Nanotechnology, with its focus on understanding and manipulating matter at the nanometer scale, is closely intertwined with the development of nano computers. Nano computers are part of the broader field of nanotechnology, where the remarkable properties exhibited by materials at the nanoscale are harnessed to create highly compact computing devices.
By operating at the nanoscale, nano computers leverage the unique characteristics of nanomaterials to achieve enhanced computational capabilities. The ability to control matter at such a minute scale allows for the creation of nano-scale transistors and components, enabling these tiny computers to perform complex data representation and manipulation tasks.
To grasp the minuteness of a nanometer (nm), it is essential to note that it represents an incredibly tiny unit of length, equivalent to one billionth (10-9) of a meter.
Just how small is a nanometer? Consider that a single human hair typically measures between 80,000 to 100,000 nm in width.
Nanomaterials can be categorized into different types using various classification methods.
Natural nanomaterials occur naturally in the environment and encompass particles found in volcanic ash, smoke, and even certain molecules within our bodies, such as hemoglobin in our blood. The vibrant colors exhibited by a peacock’s feathers, for instance, result from nanometer-scale structures on their surface.
On the other hand, artificial nanomaterials are intentionally created through human-made objects or processes. While some occur incidentally, such as exhaust emissions from fossil fuel-burning engines and certain forms of pollution, scientists and engineers actively develop nanomaterials for use across industries ranging from manufacturing to medicine. These intentionally produced nanomaterials hold promise for a wide range of applications.
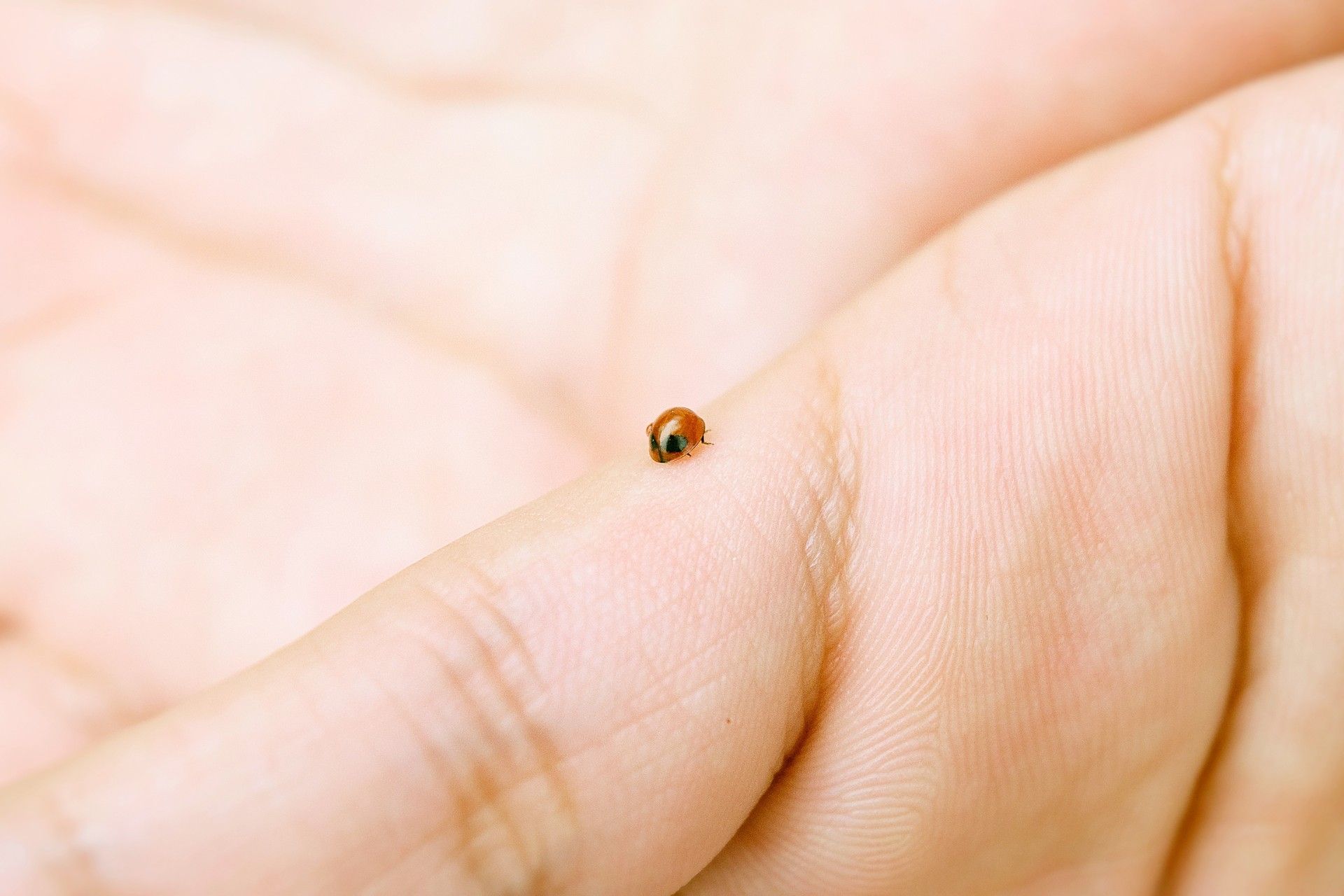
What are the different types of nano computers and how do they work?
Currently, there are several types of nano computers under development, each with its own unique approach to achieving computational tasks at the nanoscale. Here are a few notable examples:
- Molecular nano computers: These nano computers utilize individual molecules or groups of molecules as the fundamental building blocks of computation. Molecules can be engineered to exhibit specific behaviors, such as performing logic operations or storing data. By manipulating the states and interactions of these molecules, molecular nano computers can carry out computations.
- Quantum nano computers: Quantum computing harnesses the principles of quantum mechanics to process information in ways that surpass the capabilities of classical computers. Quantum nano computers rely on quantum bits, or qubits, which can exist in multiple states simultaneously, thanks to the phenomena of superposition and entanglement. Through complex quantum algorithms, these computers perform computations by manipulating qubits to explore various states and find solutions to complex problems more efficiently.
- Nano-electromechanical systems (NEMS) computers: NEMS computers integrate nanoscale mechanical components with electronic circuitry. These systems use tiny mechanical elements, such as nanowires or nanotubes, that can exhibit mechanical motion, such as vibration or bending, in response to electrical signals. By manipulating these mechanical properties, NEMS computers can perform computations based on the interaction between electrical and mechanical components.
- DNA-based nano computers: DNA, the molecule responsible for carrying genetic information, can also be used as a platform for computing. DNA-based nano computers utilize the unique properties of DNA, such as its ability to store and process information through base pairing, to perform computations. DNA strands can be engineered to encode specific algorithms or logic operations, allowing DNA-based nano computers to execute computational tasks.
The working principles of these nano computers vary depending on their design and underlying technologies. However, they typically involve manipulating and controlling nanoscale components or phenomena to carry out computational operations. This can include manipulating molecules or molecular interactions, leveraging quantum effects, utilizing nanomechanical properties, or employing DNA-based encoding and processing.
Due to their miniature size and unique properties, nano computers offer potential advantages such as high computational density, energy efficiency, and the ability to interface with biological systems. However, it’s important to note that nano computers are still in the research and development stage, and significant technical challenges need to be overcome before they become commercially viable.
Nonetheless, ongoing advancements in nanotechnology and computing continue to push the boundaries of what is possible, bringing us closer to a future where nano computers play a transformative role in various fields.
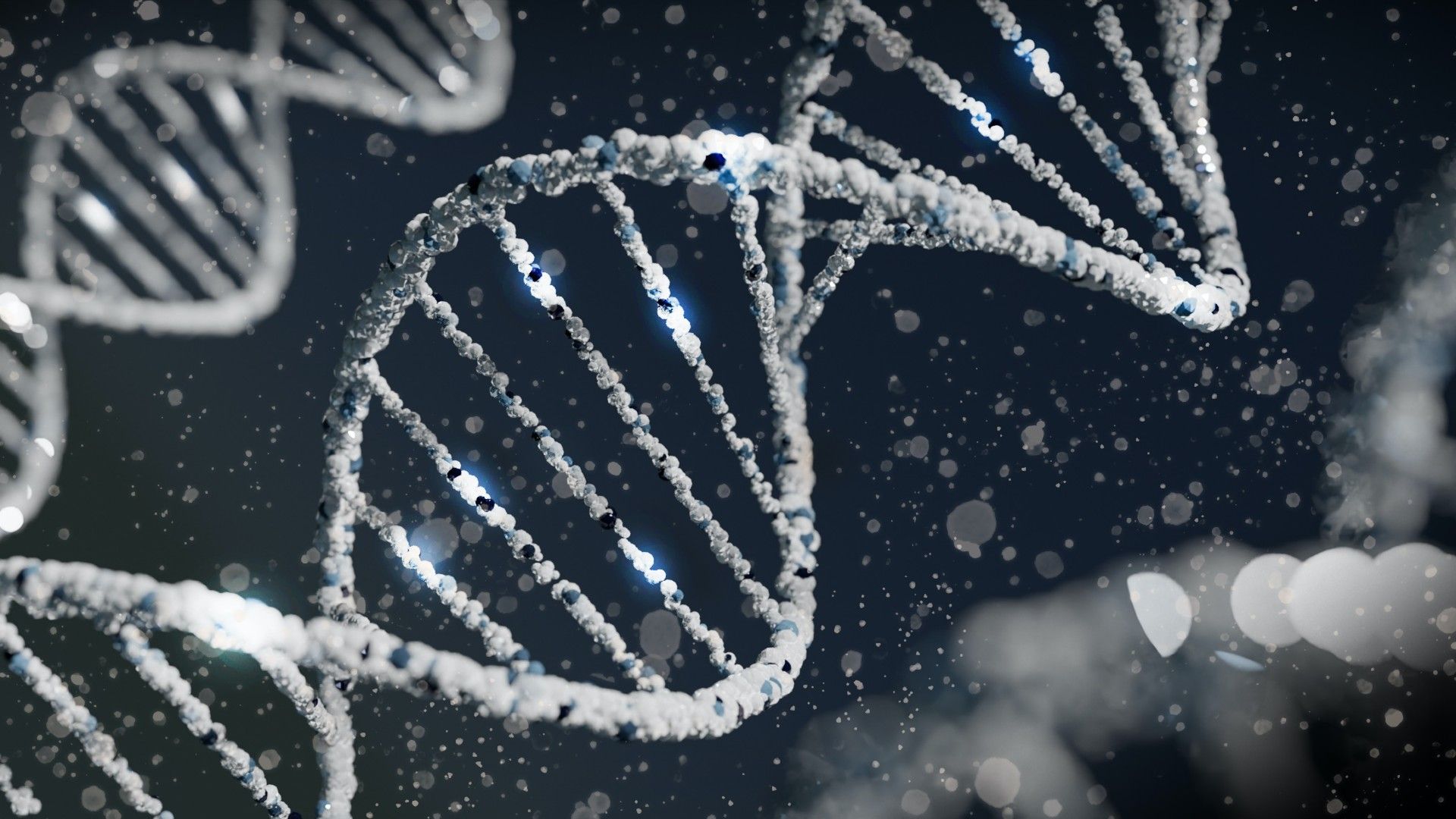
What is the difference between nanocomputing and quantum computing?
Nanocomputing and quantum computing are two distinct fields with different underlying principles and approaches. While both nanocomputing and quantum computing involves working at the nanoscale and hold promise for advancing computing technologies, they differ in their fundamental principles and goals.
Nanocomputing refers to the representation and manipulation of data using computers that are significantly smaller in size compared to traditional computers. It involves working at the nanoscale, typically at dimensions ranging from one to a few hundred nanometers. Nanocomputers utilize nanoscale components, such as nanowires, nanotubes, or quantum dots, to perform computations. They aim to maximize computational power and efficiency by taking advantage of the unique properties and phenomena that emerge at the nanoscale. Nanocomputing focuses on miniaturization and exploiting nanoscale materials to enhance computing capabilities.
On the other hand, quantum computing harnesses the principles of quantum mechanics to perform computations. Unlike classical computers that use bits to represent information as either 0 or 1, quantum computers use quantum bits, or qubits, which can exist in multiple states simultaneously, thanks to a phenomenon called superposition. This property enables quantum computers to perform parallel computations and potentially solve certain problems exponentially faster than classical computers. Quantum computing leverages quantum phenomena, such as entanglement and superposition, to manipulate and process information.
History of nano computers
The history of nano computers is a relatively recent development within the broader field of nanotechnology. While the concept of miniaturized computing has been a subject of scientific speculation for many years, significant progress toward the realization of nano computers began in the late 20th century.
One of the key figures in the early history of nano computers is American physicist Richard Feynman, often regarded as the father of nanotechnology. In his influential 1959 talk titled “There’s Plenty of Room at the Bottom“, Feynman discussed the potential for manipulating and controlling individual atoms and molecules. Although he did not explicitly use the term “nano computers”, his ideas laid the groundwork for the field.
The advent of the scanning tunneling microscope in 1981 marked a crucial milestone in the development of nano computers. This revolutionary instrument, invented by IBM scientists Gerd Binnig and Heinrich Rohrer, enabled researchers to observe and manipulate individual atoms with unprecedented precision. Binnig and Rohrer’s groundbreaking work with the scanning tunneling microscope earned them the Nobel Prize in Physics in 1986 and paved the way for further advancements in nanoscale research.
In the following years, researchers and engineers made significant strides in nanotechnology, leading to the emergence of nano computers. The ability to manipulate and control nanoscale components and materials became a reality, fueling the vision of highly compact computing devices with remarkable computational capabilities.
A notable milestone in the history of nano computers occurred in 1990 when IBM researchers successfully demonstrated the world’s smallest computer at the time, measuring a mere 3x3x4 millimeters. This achievement showcased the potential for creating functional computing systems at the nanoscale.
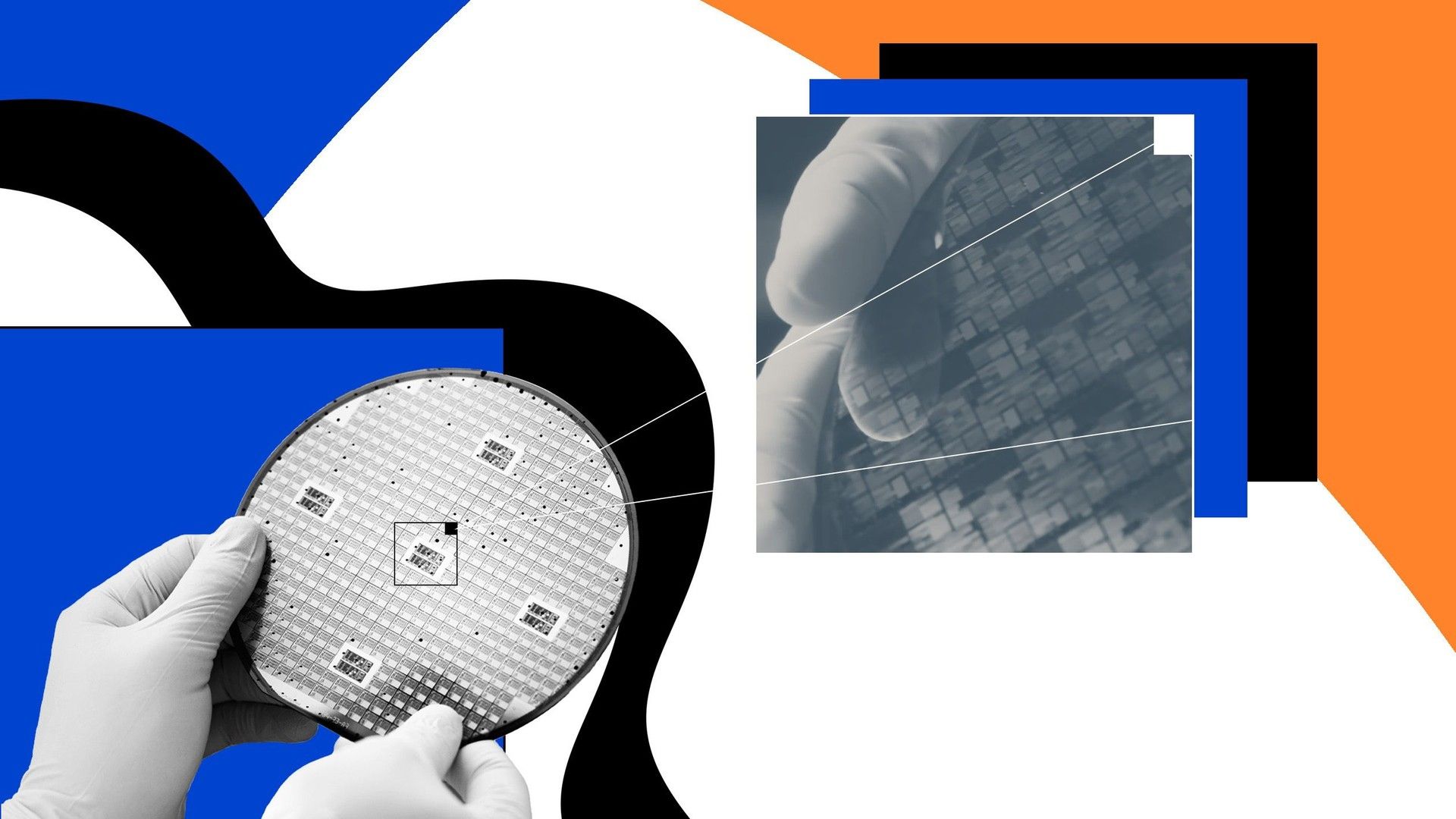
Since then, ongoing research and development have pushed the boundaries of nano computing. Scientists have explored various approaches, including the use of nanoscale transistors, quantum effects, and novel materials, to build increasingly powerful and efficient nano computers. The field has seen advancements in areas such as nanomaterial-based logic circuits, nanowire networks, and molecular-scale computing architectures.
While nano computers are still in the early stages of development and face significant technical challenges, they hold immense promise for the future of computing. These miniature computing devices offer the potential for unprecedented computational power, energy efficiency, and integration into diverse applications, ranging from healthcare and electronics to environmental monitoring and beyond.
What are the benefits of using nano computers?
While the development of fully functional nano computers is still a subject of ongoing research and exploration, there are several potential benefits that could be associated with their use.
Size and portability
Nano computers would be incredibly small in size, with dimensions measured on the nanometer scale (1 billionth of a meter). Their miniature size would allow for unprecedented portability and integration into various objects and systems, enabling applications in fields such as medicine, electronics, and environmental monitoring.
Tiny but powerful
Nano computers could potentially offer a significant increase in computing power compared to traditional computers. With advancements in nanotechnology, these devices might be able to pack a substantial amount of processing power into an incredibly small form factor. This enhanced computing capability could lead to more efficient data processing, complex simulations, and advanced calculations.
Key to energy efficiency
Nano computers could potentially operate at extremely low power consumption levels. Due to their miniature size, they would require minimal amounts of energy to function, making them highly energy-efficient. This aspect could have positive implications for battery life and energy sustainability, enabling longer device usage without frequent recharging or battery replacement.
Nanomagnets will pave the way for low-energy AI
Improved sensing and data collection
Nano computers could be equipped with advanced sensing capabilities, enabling them to gather data at unprecedented levels of precision and accuracy. Their miniature size could allow them to be deployed in environments that are inaccessible to larger devices, opening up new possibilities for monitoring and data collection in areas such as healthcare, environmental sensing, and industrial applications.
Integration into complex systems
The small size of nano computers would make them well-suited for integration into complex systems, including biological systems. They could potentially be used for targeted drug delivery, real-time monitoring of physiological parameters, or even as components of artificial organs. This integration could revolutionize fields such as medicine and bioengineering.
The development and realization of nano computers are still within the borders of scientific speculation and theoretical research. While the potential benefits are intriguing, practical implementation and overcoming the numerous technological challenges remain significant hurdles to overcome.
Why we have not yet entered the nano-age?
Nano computers, despite their immense potential, face several significant challenges that need to be overcome for their successful development and integration into various applications.
One of the primary challenges lies in the manufacturing process at the nanoscale. Fabricating nanoscale components with high precision and consistency, while ensuring scalability and cost-effectiveness, is a complex task. The development of reliable and efficient manufacturing techniques for nanoscale transistors, interconnects, and other components is crucial for the mass production of nano computers.
Another challenge is ensuring the reliability and stability of nanoscale devices. At the nanoscale, phenomena such as quantum effects and surface interactions become more pronounced, impacting the performance and longevity of nano computers. Addressing issues related to material degradation, noise, and environmental conditions is essential for maintaining the reliability and stability of nanoscale components over extended periods.
Heat dissipation and energy efficiency are also critical challenges in the development of nano computers. As these devices continue to shrink in size and operate at higher computational speeds, managing heat becomes increasingly difficult. Efficient heat dissipation mechanisms need to be devised to prevent overheating and ensure the long-term reliability of nano computers. Additionally, enhancing energy efficiency is crucial to meet the growing demand for computational power while minimizing power consumption.
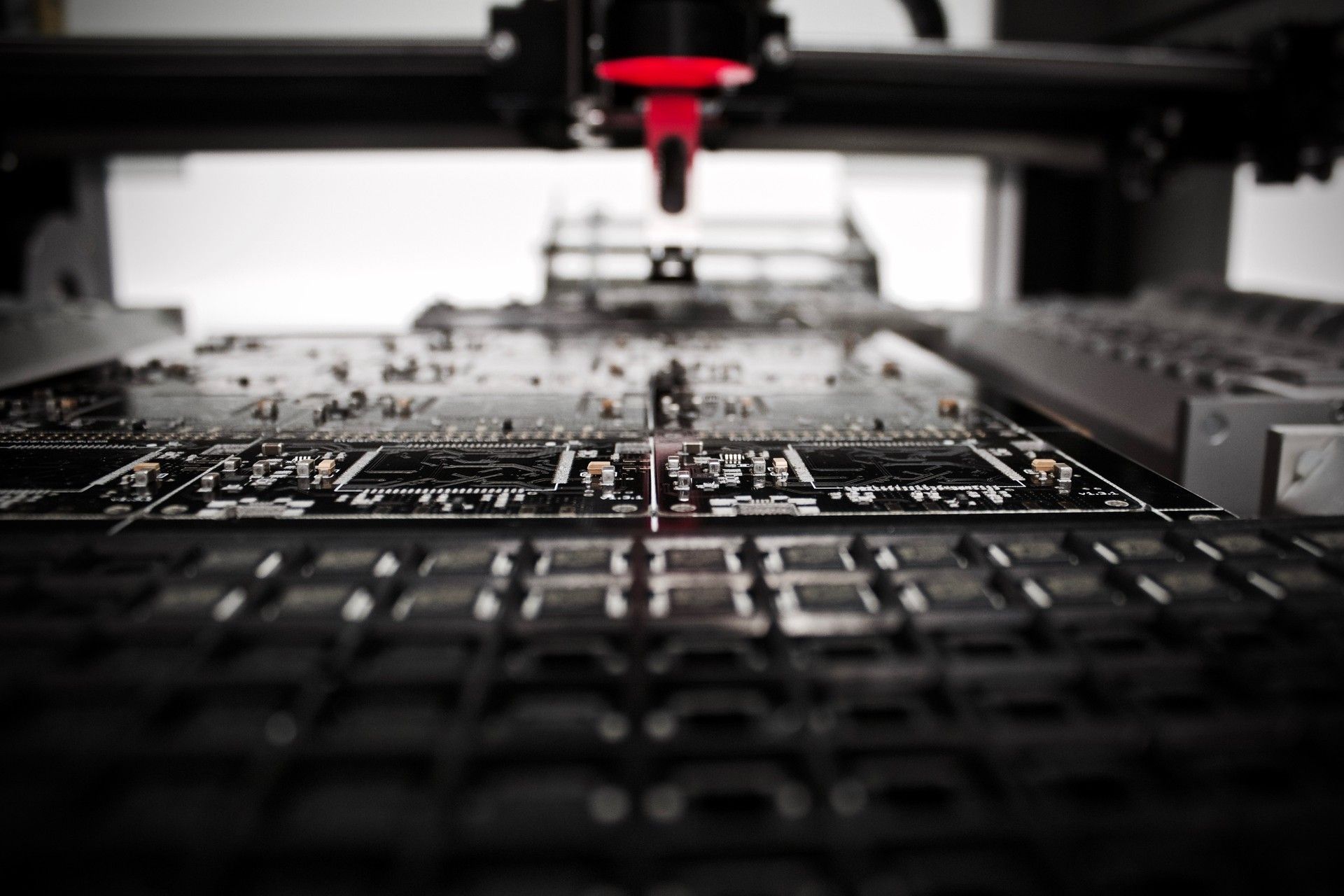
The integration of nano computers with existing technologies and systems poses another significant challenge. Seamless compatibility, efficient interconnectivity, and the design of interfaces that enable smooth communication with other devices and networks need to be addressed. Developing effective ways to interface nanoscale components with macro-scale systems and infrastructure is essential for the successful integration of nano computers into various applications.
The use of nanomaterials in nano computers raises concerns about their potential toxicity and environmental impact. Understanding the health and safety implications of nanomaterials, as well as their long-term effects on ecosystems, is crucial for responsible development and deployment. Robust regulations and guidelines should be put in place to ensure the safe use and disposal of nanomaterials in nanocomputing technologies.
Cost and scalability are also major challenges. The manufacturing cost of nano computers needs to be reduced to make them commercially viable, while scaling up production processes without compromising quality and reliability is essential for meeting market demands. Finding cost-effective fabrication methods and establishing efficient scaling strategies are key to the widespread adoption of nano computers.
Lastly, ethical and societal implications must be considered. Protecting sensitive data, ensuring cybersecurity, and addressing potential impacts on employment and equitable access to nano computing technologies require careful attention and proactive measures.
Nano computers, which will completely change the future and the way we approach most areas of science, are pushing the limits of human creativity and imagination, competing with artificial intelligence technologies to become one of humanity’s most important inventions.